ESP32 precision GPS receiver (incl. RTK-GPS Tutorial). How to earn money with it (DePIN)
Summary
TLDRفي هذا الفيديو، نتعرف على تقنية نظام الملاحة ال衛ي الشاملة (GNSS) وكيفية استخدامها لتحديد مواقع بدقة高达公分级别. يُظهر لنا القناة كيفية استخدام موديلات RTK لتحسين دقة نظام GPS التقليدي، مما يتيح لنا القدرة على قياس المسافة إلى ال衛ي المتحركة بسرعة واستخدام هذه المعلومات لحساب مكاننا الدقيق. نرى أيضًا كيف يمكن للتكنولوجيا هذه أن تساعد في بناء قاعدة بيانات عالمية وكيفية الربح من خلال إنشاء وتوصيل قاعدة بيانات خاصة.globally networked base station. كما نتعلم عن DePINs وكيف يمكن للناس الاستفادة الاقتصادياً من هذه التكنولوجيا.
Takeaways
- 📡 تمكن تقنية نظام الملاحة ال衛성ية الشاملة (GNSS) من قياس المسافة بدقة高达 3 متر باعتماد تقنية قياس الزمن بدقة高达 10 نانو ثانية.
- 🛰️ تتطلب الدقة الأعلى (المركزي) قياس زمن الإشارات بدقة高达 100 بيكوس وتعويض لأخطاء المواقع ال衛성ية高达 السنتيمتر.
- 🔍 يستخدم GNSS لتحديد الموقع عن طريق قياس زمن إشارة ال衛星 المرتقبة، والتي يمكن أن تكون ضعيفة جدًا بسبب المسافة البعيدة.
- 📊 يستخدم التكرار الرقمي العشوائي (الكود الوهمي) من قبل ال衛星ات ل允許 لاستقبالها بواسطة الاستقبالات ال衛성ية.
- ⏱️ لتحسين الدقة، يمكن استخدام تقنية RTK (العلم الكيني الحي)، التي ت对比 بين الاستقبالين الثابتة والمتحركة لتحديد الاختلاف.
- 💡 يمكن للخدمات الصناعية الأساسية أن تبيع إشارات تصحيح RTK بأسعار عالية، بينما تحاول شركات DePIN مثل Onocoy تقليل الأسعار.
- 🌐 يمكن للمستخدمين المساهمة في شبكة عالمية من الاستقبالات الأساسية والحصول على ال加密货币 كأجر عن خدماتهم.
- 🛠️ يمكن بناء الاستقبال الأساسي بتكلفة أقل من الاستقبالات التجارية التقليدية، مع القدرة على التوصيل مع خدمات مثل RTK2GO أو Onocoy.
- 📍 يساعد RTK في تحسين الدقة المكانية بشكل كبير، مما يتيح تطبيقات متعددة مثل الطيران اللاسلكي والجرافات الصناعية.
- 🔗 يمكن لل衛성ات ال衛성ية المساعدة في تحسين الدقة من خلال تزويدي الاستقبالات ال衛성ية بمعلومات إضافية عن مسار ال衛ست.
- 💻 يمكن للأجهزة الذكية استخدام تقنية GPS مساعدة عبر الإنترنت لاسترداد الموقع بشكل أسرع بالإشارة إلى البيانات المطلوبة مباشرة من الإنترنت.
Q & A
هل من الممكن تحديد الموقع بدقة تصل إلى السنتيمتر حول العالم؟
-نعم، من الممكن، كما يشير النص إلى أنه يمكن تحقيق هذا الدقة الفائقة باستخدام ESP32 وجهاز مستقبل GPS جيد.
ماذا يشير النص بـ 'DePINs'؟
-'DePINs' يشير إلى 'decentralized physical infrastructure networks'، وهي شركات تحاول استبدال الاستثمارات بتمويل جماعي وإنشاء عملة إلكترونية لدفع مالكي البنية التحتية.
كيف يعمل نظام GPS في قياس المسافة إلى ال衛星 المتحركة بسرعة؟
-يعمل نظام GPS عن طريق قياس زمن إشارة ال衛星 بدقة高达 10 نانو ثانية لكي يتمكن من حساب المسافة بدقة高达 3 متر.
ماذا تعني RTK في سياق النص؟
-RTK تعني 'real-time kinematics'، وهو تقنية تستخدم لتحسين دقة GPS من قياسات بضعة المتر إلى السنتيمتر.
ما هي الخطوات الأساسية لبناء محطة قاعدة RTK؟
-لإنشاء محطة قاعدة RTK، تحتاج إلى مستقبل RTK شامل، ESP32، وبرنامج لقراءة البيانات من الاستقبال وإرسال إشارات ال校正 إلى الخدمة.
كيف يمكن لشخص يستخدم RTK أن يكسب المال؟
-يمكن لشخص يستخدم RTK أن يكسب المال عن طريق بناء محطة قاعدة وتوصيلها بشبكة عالمية مثل Onocoy، التي تشتري البيانات بالعملة الرقمية.
ماذا تشير إلى الجملة 'The width of peak is about 1ns or 30 meters'؟
-تشير إلى أن عرض الذروة في الرسم البياني الذي يظهر تطابق نمط الإشارة القادمة من ال衛星 مع نمط الكود المعروفة هو حوالي 1 نانو ثانية، مما يعادل 30 متر في الدقة.
لماذا يتطلب نظام GPS ال衛star ال-clocks ال precisos؟
-يتطلب الـ GPS الـ clocks ال precisos لضمان تطابق زمني بال衛star البعيدة، مما يسمح بدقة قياسات المسافة وتحديد المواقع بدقة.
ما هي العوامل التي تؤثر على دقة نظام GPS؟
-العوامل التي تؤثر على دقة نظام GPS تشمل تأخيرات الاتزان الجوي، تأثيرات اليونوسفر، ودقة الـ clocks في ال衛star والاستقبال.
كيف يمكن لنظام RTK التعامل مع مشكلة الـ 'ambiguity'؟
-RTK يحل مشكلة الـ 'ambiguity' من خلال مقارنة نمط الكود القادم من ال衛star مع نمط الكود المعروفة لتحديد بدقة المكان على الموجة ال несائية.
ماذا يشير النص بـ 'almanac' في سياق GPS؟
-في سياق GPS، يشير 'almanac' إلى بيانات بسيطة تتضمن معلومات حول ال衛star مثل موقعها والاتجاهات، التي يتم استلامها من ال衛star وتستخدم لتحديد المواقع بشكل أسرع.
Outlines
📍 GPS Precision and Global Networks
The first paragraph introduces the concept of achieving centimeter-level location accuracy globally using an ESP32 and a GPS receiver module. It discusses the possibility of earning money by building a base station and connecting to a global network. The speaker expresses excitement about the technology and invites viewers to learn about how GPS measures distances to satellites to calculate exact positions. It also touches on the history of GPS, its initial accuracy limitations, and the development of other satellite systems like GNSS.
🚀 Understanding GNSS and RTK Technology
The second paragraph delves into the technicalities of how GNSS works, focusing on the importance of precise time measurements for distance calculations. It explains the challenges of decoding weak signals and distinguishing between different satellite signals. The concept of 'cross-correlation' is introduced to describe how GPS receivers identify signals from specific satellites. The paragraph also outlines the need for atomic clocks in satellites and receivers, and a clever method to overcome the limitations of less precise receiver clocks.
🔍 Enhancing Precision with RTK
The third paragraph discusses the limitations of standard GPS precision and introduces Real-Time Kinematics (RTK) as a solution to achieve higher accuracy. It explains the RTK system, which involves a fixed 'base' receiver and a movable 'rover' receiver, and how they use correction signals to improve positioning data. The paragraph also explores various methods for transmitting correction signals, including direct transmission, internet, mobile networks, and satellite uplinks.
🛠 Building a DIY RTK Base Station
The fourth paragraph provides a practical guide on building a rover using an RTK-enabled GPS receiver and an ESP32. It mentions the potential to earn money by creating a base station and selling correction data, introducing the concept of Decentralized Physical Infrastructure Networks (DePINs). The speaker shares their experience with building a base station, connecting it to RTK2GO, and the cost benefits compared to commercial options. It also briefly touches on how GPS satellites synchronize clocks and their importance in cellular networks.
🌐 Commercial Services and Personal Investment
The final paragraph summarizes the key learnings from the video, emphasizing the need for precise time signals in GPS and the role of at least four satellites in determining a three-dimensional position. It highlights the limitations of GPS accuracy and how RTK can enhance precision to the centimeter level. The speaker shares their decision to build a base station and partner with Onocoy, a DePIN company, to provide correction signals globally. The video concludes with an invitation to support the channel and a promise to provide relevant links for further information.
Mindmap
Keywords
💡GNSS
💡RTK
💡Phase
💡DePIN
💡Cross-correlation
💡Almanac
💡Ionosphere
💡Carrier wave
💡Base station
💡Rover
Highlights
It is possible to determine your location to the centimeter around the globe using an ESP32 and a GPS receiver module.
Building your own base station and connecting it to a global network can potentially earn you money.
The video provides a clear understanding of measuring distances to satellites and calculating exact positions using this information.
The technology enhances standard GPS and can deliver centimeter precision through a DIY ESP32 base station.
GPS was invented in the 1970s and became more accurate after the US military stopped artificially degrading its signals post Gulf War.
GNSS, including GPS, GLONASS, and Galileo, is a network of satellite systems providing global navigation.
Measuring time with a precision of 10 nanoseconds allows for a distance measurement precision of 3 meters.
GPS receivers use cross-correlation to identify signals from specific satellites and their precise timing.
All satellites have built-in atomic clocks regularly adjusted by ground stations to maintain synchronization.
RTK (Real-Time Kinematics) is a method that allows for centimeter-level positioning accuracy by using a fixed base station and a rover.
The base and rover can communicate corrections via direct transmission, internet, mobile networks, or satellite.
The ESP32 board includes Wi-Fi and a 4G modem for connecting to cellular networks and receiving satellite data.
RTK-enabled GPS receivers and an ESP32 can be used to build a rover for precise positioning.
DePINs (Decentralized Physical Infrastructure Networks) are networks that use cryptocurrency to incentivize infrastructure owners.
Onocoy is a DePIN company aiming to build a global network of base stations for RTK correction data at a lower cost.
Building a base station requires a special antenna, an RTK receiver, an ESP32, and software to send correction signals.
The video demonstrates the construction and operation of a DIY base station connected to RTK2GO for enhanced GPS precision.
The presenter's setup cost less than $300 and has already started to earn cryptocurrency by providing RTK correction data.
The video concludes by emphasizing the importance of GPS synchronization for various technologies and the potential threat of signal jamming.
Transcripts
Determine your location to the centimeter around the globe? For everybody, not only for
the military? Only with an ESP32 and a decent GPS receiver module? Not possible! Wrong,
it is possible. You can even earn some money by building your own base station and connecting it
to a global network! When I got this board from Michael, a viewer of this channel,
I was hooked on the idea of trying and understanding this relatively new technology. What
about you? Interested, too? Then, follow along. Grüezi YouTubers. Here is the guy with the Swiss
accent. With a new episode and fresh ideas around sensors and microcontrollers. Remember: If you
subscribe, you will always sit in the first row. By the end of this video, you'll have a clear
understanding of how it's possible to measure the distance to fast-moving satellites more than
20,000 kilometers away and use that information to calculate your exact position. You'll also
see how this technology enhances standard GPS. To demonstrate, we'll use my DIY ESP32 base station,
connect it to a global network, and see if it can really deliver centimeter precision. And finally,
learn what DePINs are and how you can earn money with this technology. This isn't just about the
technology, it's about the practical applications and the potential it holds for us all.
GPS was invented in the 1970s and implemented from the 1980s on. In 1995, I had my first
Garmin handheld GPS receiver for pilots. It was mind-boggling because from now on, I always
knew where I was and where the forbidden zones started. A big stress reliever! The accuracy of
this degraded non-military system was around 100 meters. Useful for pilots because they use wide
“airways”, but not yet for car drivers. After the gulf war, the US military stopped the artificial
degradation of the GPS signals and so improved its accuracy. I strongly suggest reading or listening
to “You Are Here” if you are interested in how it all began. Other nations like the Chinese,
the Europeans, and the Russians started to build their own “GPS” systems. Together, they are called
“Global Navigation Satellite System”, short GNSS. How does GNSS work? I will use GPS to explain it.
The most important fact is: In one microsecond, light and also radio waves travel about 300
meters and 30 cm in one nanosecond. If we want to measure the distance with
a precision of 3 meters, we have to be able to measure time with a precision of 10 nanoseconds,
and if we want to measure 3 cm, we need to get to a precision of 100
picoseconds. Not bad. Keep in mind: These satellites are 20’000km away,
move at high speed, and have to have exactly the same synchronized time. At first glance,
this seems to be impossible! But let’s see how they managed to make it work.
To determine its position, a GPS receiver needs to calculate the distance to at least four satellites
by measuring the timing of the signals. For that, it listens to 1575.42MHz or L1, where
all GPS satellites transmit their signals. In the meantime, other frequencies were added, mainly L2
at 1227.6MHz and L5 at 1176.45MHz. The engineers back in the 1970s had to solve two main problems:
1. How to decode very weak signals traveling 20’000 km through space?
2. How to distinguish between signals of different satellites on the same frequency?
Let’s monitor these three frequencies with a Spectran SDR receiver. All contain more or
less random noise with no visible carriers. Interesting! If I connect the same antenna
to a proper GNSS receiver module, it shows my position. Obviously, there are “hidden” signals
on these frequencies. How does this work? Back then, they decided that each satellite
transmits its own “pseudo-random” pattern of 1023 bits with a rate of 1.023Mbit/s. That
is the reason we can hardly distinguish them from noise. Because these patterns are known
to all GPS receiver modules, they can compare the signals coming from all satellites with all
known patterns. Mathematicians call this process “cross-correlation”. The result is a peak when
the received signal pattern and the code of one particular satellite match in time. Nearly no peak
is visible for signals of other satellites. So, such a peak contains two parts of information:
1. Which satellite sent the signal 2. Its precise timing
The width of peak is about 1ns or 30 meters. Even if you can determine the peak very accurately,
the precision of this signal is limited to a few meters because there are other sources of
inaccuracies, as we will later see. This is the precision of our smartphones, for example. After
receiving the signals of all visible satellites, our GPS receiver knows the distance to these
satellites. But only if the clocks of all satellites and our receiver are exactly
synchronized. Keep in mind: A difference of one nanosecond means already an error of 30 meters!
This is why all satellites have built-in atomic clocks that are regularly adjusted by ground
stations. Our GPS receiver module has a clock, too. But to save cost and space, not a very
precise one. So, the whole thing would not work unless we use a trick that is later revealed.
The next problem: To get our precise position, we need not only the distance to the satellites;
our receiver also needs to know the momentary position of each satellite,
also with the precision of meters. We will later see where it gets this information from.
To calculate a three-dimensional position, the distance to at least three satellites
and their precise positions are needed. The trick to working with the unprecise receiver
clock is to use the signal of a fourth satellite to calculate the precise time.
Now, we are ready to retrieve the exact position with a precision of a few meters
everywhere on Earth. It's incredible, but it works with a receiver module for
a few dollars and such tiny antennas. As said before: We want more. 100 times
more precision. Sounds impossible again! Let’s try to understand which problems we
have to solve to get to such a precision: 1. We have to be able to measure the travel
time of the signal to the picosecond 2. We have to compensate for position
errors of satellites to the centimeter 3. We have to account for time delays
influenced by the ionosphere. The ionosphere is the upper part of the atmosphere and consists of
charged particles. They are heavily influenced by the sunlight and therefore change all the time
4. And correct many more small errors in the overall system
Let’s start with the first problem: Increase the precision of the timing. As we saw before, GPS has
a modulation frequency of 1Mb/s. But its “carrier” frequency is around 1.5GHz and, therefore,
a wavelength of around 20 cm. What if we would be able to determine where on this wave we are? Then,
we would know our position to the centimeter! Technically, the place on a wave is called
“phase”, BTW. Problem solved? Unfortunately, not. As shown before, GPS has a precision of
some meters. Let’s assume a precise GPS position of 5 meters. Then, 20 wavelengths fit inside these
5 meters. This is rightly called ambiguity because we know exactly where we are on the wave but,
unfortunately, not on which one. Not good! Clever engineers developed real-time kinematics or
RTK to solve this problem: Let's assume you have two receivers close together. One is fixed, and
its position is exactly known; the other can be moved, and its position is not known. Both measure
the position with GPS and determine their phase. Because one knows exactly where it is, it can
determine on which wave it “sits” and determine the actual difference between its position
and the GPS position. If it would transfer this information to the second receiver, this one could
determine its exact position, too. And we solved problems 2, 3, and 4. Because both receivers are
very close, all these differences are nearly the same and are included in the “correction”
signal transmitted. Cool! If we call the fixed receiver “base” and the second one “rover”,
we have our RTK system. Of course, it is way more complex, but for today, we stick with that.
Fortunately, the distance between the base and the rover can be up to about 20 km,
and the system still works. The next problem: How is this
correction signal transmitted from the base to the rover? Here, we have four typical scenarios:
1. Directly by using a transmitter on the base and a receiver on the rover. High-end lawn movers
attach a base to the charging station and the rover to the mover. Also high-end drones work with
fixed bases close to the pilot. In this scenario, each rover needs a base station. Commercial base
stations, unfortunately, cost a fortune 2. Via internet. The base and the rover are
connected to a service. The base transmits the correction signal to the platform,
and the rover receives a valid correction signal without “owning” a
base. Signals can be transmitted via Wi-Fi 3. Or via mobile networks. There are many
such professional services available. Usually very local and very expensive
because building and maintaining bases every 20 km is not cheap. RTK2GO is a free service,
but it only works if you have a base in the vicinity. The closest one to my home is 40
km away. So later, I will build my own for a fraction of the price of a commercial one
4. Via satellite. Companies like u-blox operate many base stations around the
world. Because they cannot afford one every 20 km, they placed them about 150km apart and
do some math to their signals. Like that, they typically get a precision of below one meter,
but not to the centimeter. Still ok for many use cases and available globally. But not cheap.
The board I got from Michael offers transmission methods 2, 3 and 4. The ESP32 includes Wi-Fi,
and this 4G modem can connect to the next cellular tower. It even
contains a satellite receiver that can receive u-blox data from space. They offer a limited
service for developers free of charge, BTW. It also contains this small u-blox RTK receiver
that covers the most important bands, L1 and L2, and shows its position on a map. So,
let's check how it works. As said before, I wanted the best precision. So I built a
base station and connected it to RTK2GO. We go away from the house to reduce signal
reflections and start with GPS only. As expected, the position moves a few meters. With RTK enabled,
this changes considerably. The position is solid. And if I move the receiver, or should I say,
the antenna, along a straight line, we see this straight line also on the map. Impressive!
Here, you see typical applications for RTK. Maybe something is for you?
To build a rover, we just need an RTK-enabled GPS receiver and an ESP32 if we have Wi-Fi available,
plus Michael’s Arduino software. Sparkfun offers many such receiver modules and also
wrote the required libraries. We know now that RTK works,
and we saw this rover board. But I also promised that you could earn money with RTK. How does that
work? And how can we build a base station? Helium, with its network of LoRaWAN gateways,
was one of the first companies that created a new industry called “decentralized physical
infrastructure networks”, short DePIN. DePIN companies try to replace investors
with crowdfunding. Like Helium, they create a cryptocurrency and pay the owners of the
infrastructure with this currency instead of real money till they get paid by the customers.
Everybody who had to work with professional investors knows that this would be a very good
idea for startups because often, these “investors” are arrogant and a pain in the ass. People who
watched my Helium video know that I was not happy about this company. Mainly because there was no
real business behind transferring LoRa messages. But is DePIN in general a bad idea? I do not
know yet because it is too new. But I give it a chance if there is a real business case behind
it. Selling RTK correction data seems to be a multi-million-dollar business already now. So this
market should be better than LoRaWAN messages. And its future application is much broader.
A few DePINs have already tried to get such networks up and running. One of them is
Onocoy. This network has two advantages: 1. They allow Makers to create their own
hardware and do not sell overpriced “miners” 2. Its president is one of the founders of u-blox,
a no-nonsense guy also with a Swiss accent How can we build such a base station? You need
four things to get the best signal and, therefore, the most rewards:
- A special antenna for all GPS bands - An RTK receiver for all bands
- An ESP32 - Software to read the receiver
and send the correction signal to the service You can buy this receiver with an ESP32, but it is
expensive and only delivers data to Onocoy. This is why I built one myself. Mine delivers data to
Onocoy and RTK2GO. I do not show how to build it here, but you can find links in the description if
you are interested in this technology. Anyway, connecting four wires between the receiver and
the ESP32 board and loading the software is all it needs. The rest is configuration.
Keep in mind: Commercial stations cost thousands of dollars. This miner still costs roughly
700 dollars, including shipping. My setup was less than 300 dollars. It's not cheap, but maybe I will
get the money back or make more than I invested. It has already started to earn some cryptos.
Even if I will not earn a lot, I had a good time learning and experimenting with this
technology. It helped me understand one of the key technologies of our civilization, and I pay
respect to the engineers who, more than 40 years ago, believed that such a system was possible and
started to work on it. Keep also in mind that GPS satellites synchronize most of our clocks and are
part of each cellular tower, for example. I made a video on how you can use this precise timing
for cheap in your lab. Jamming these signals seems to be a major threat during war times.
I also promised to tell you how GPS receivers get the positions of all satellites. The pseudo-random
code contains a very slow modulation that transfers all this data, also called “almanac”.
It can take more than 10 minutes to get all this data. This is why all GPS modules have a small
battery attached. They store the almanac data and, because satellites do not frequently change their
path, can use “old” data to get a faster fix. If the GNSS receiver has an internet connection,
like a Smartphone, it uses “assisted GPS” to get this information much faster directly via
the internet. This is why your Smartphone nearly immediately knows its position.
In this video: - We learned that GPS
needs extremely precise and synchronized time signals to measure the distance
between satellites and our receiver - It needs at least four satellites,
three to determine the three-dimensional position and one to correct the time of the receiver
- Its accuracy is limited by the slow modulation as well as by variable
influences by the ionosphere, for example - If we want to get to centimeter precision,
we need to measure the phase of the carrier signal as well as account for the real-time difference
between a precise position and the signal - This difference is measured by a base station
and transmitted to a “roving” station to enable the rover to solve the ambiguity and determine its
exact position. The distance between a base and a rover should be below 20 km to get an RTK fix
- Commercial services sell such correction signals for quite high
prices. RTK2GO offers a free-of-charge service but does not have broad coverage
- This is why I built a base and connected it to Onocoy, a DePIN company that tries to
build a global network of base stations and sell the data for a lower price. I will be
paid in cryptocurrency for my services. Who knows if I will get the invested money back
This was all for today. As always, you find all the relevant links in the description.
I hope this video was useful or at least interesting for you. If true,
please consider supporting the channel to secure its future existence. Thank you! Bye
5.0 / 5 (0 votes)
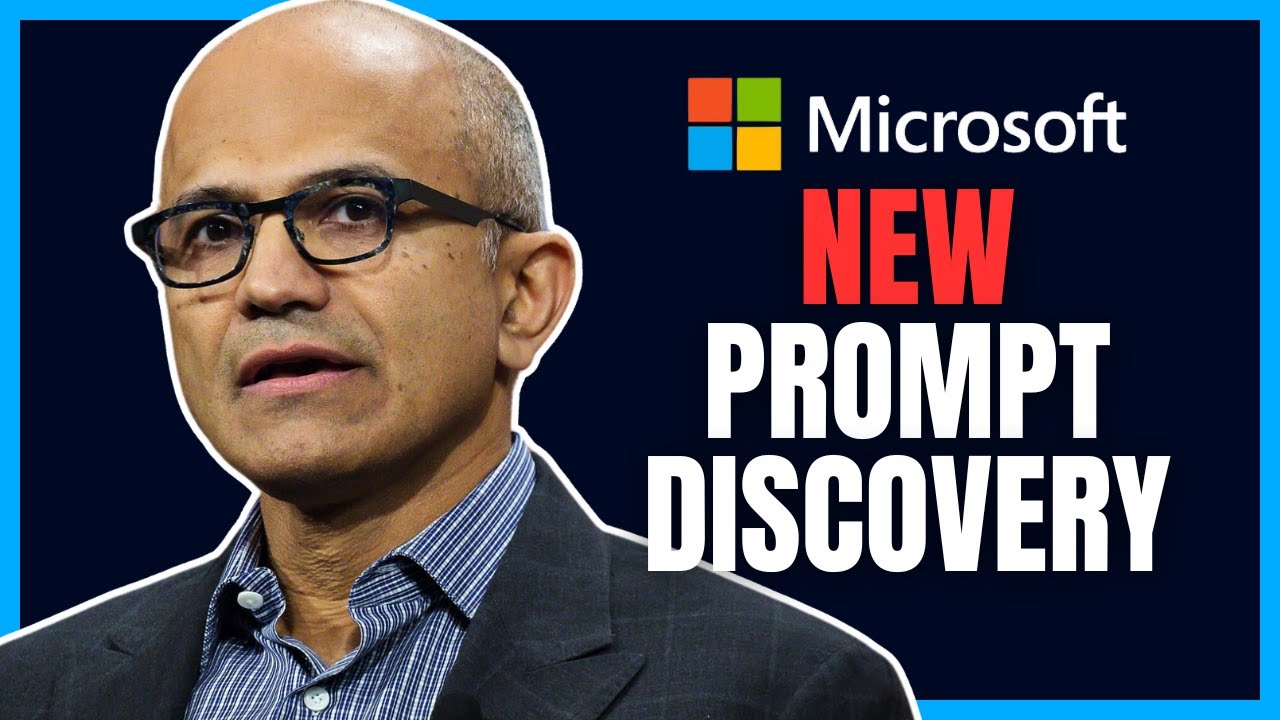
"VoT" Gives LLMs Spacial Reasoning AND Open-Source "Large Action Model"
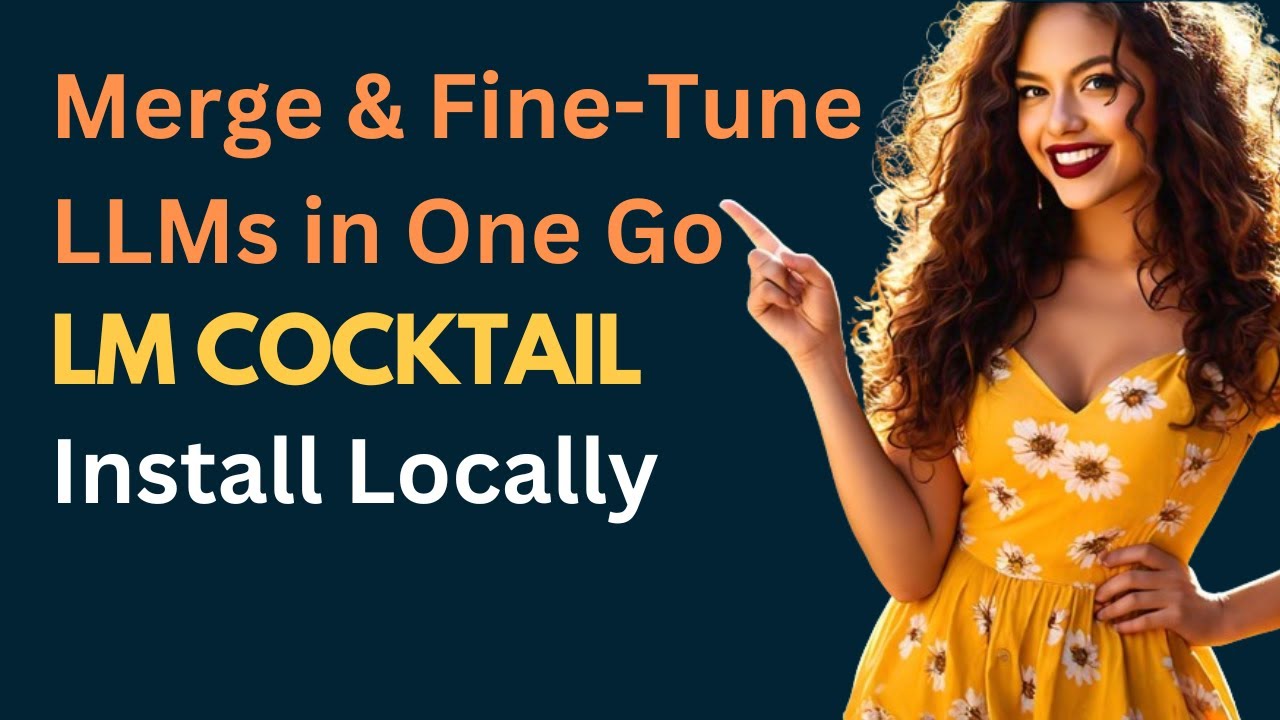
Merge Models Locally While Fine-Tuning on Custom Data Locally - LM Cocktail
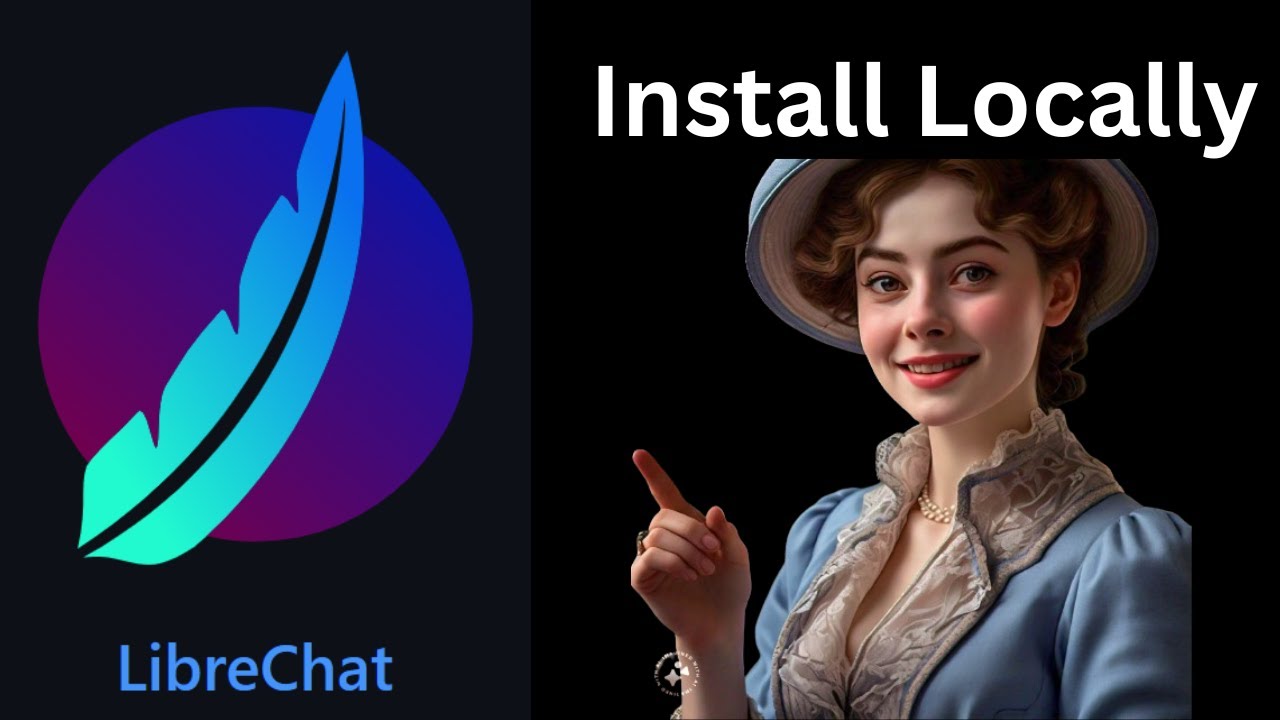
Install LibreChat Locally
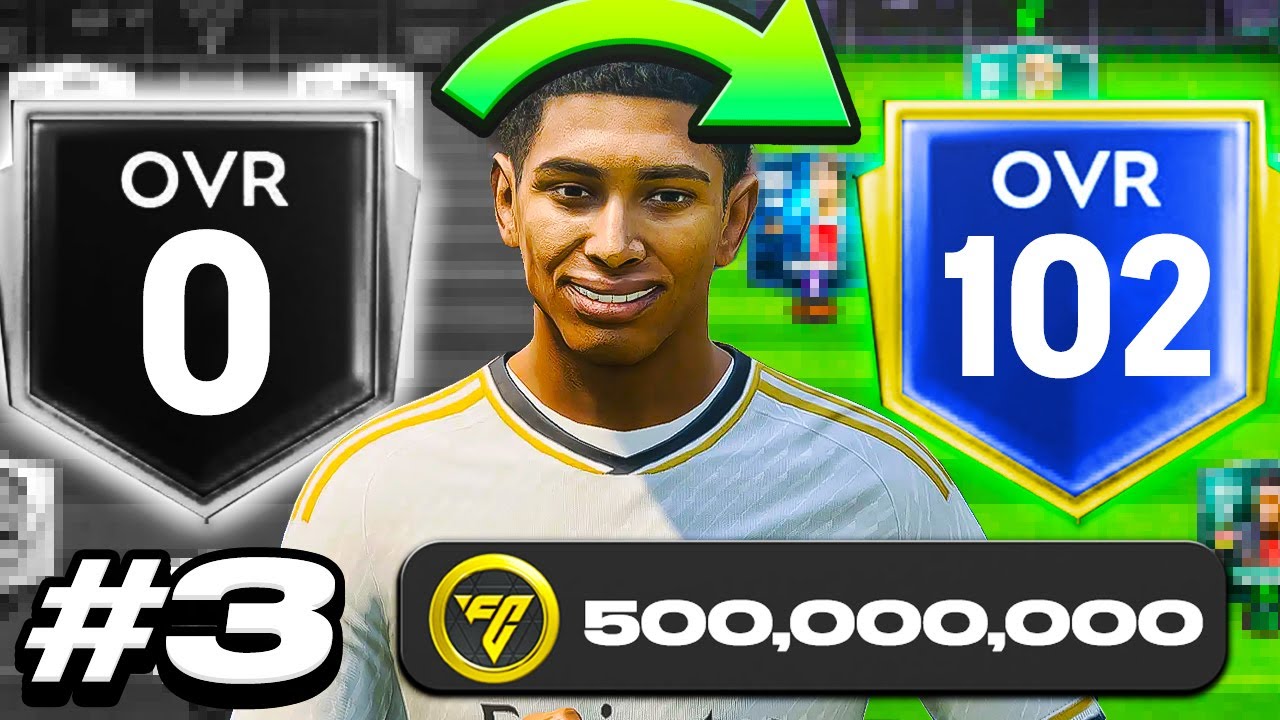
450 Million Coins Made & Huge Squad Upgrade - 0 to 102 BROKE FC (Episode 3)
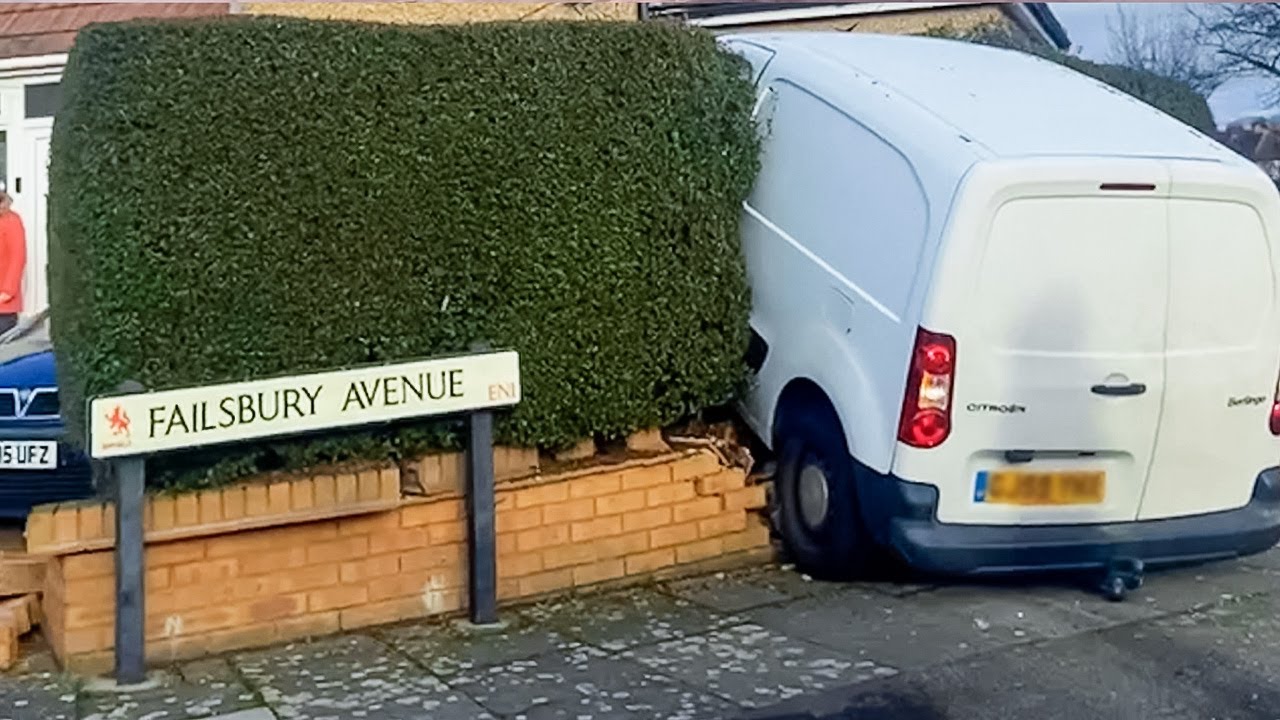
When Everything Goes Wrong | Funny Fails
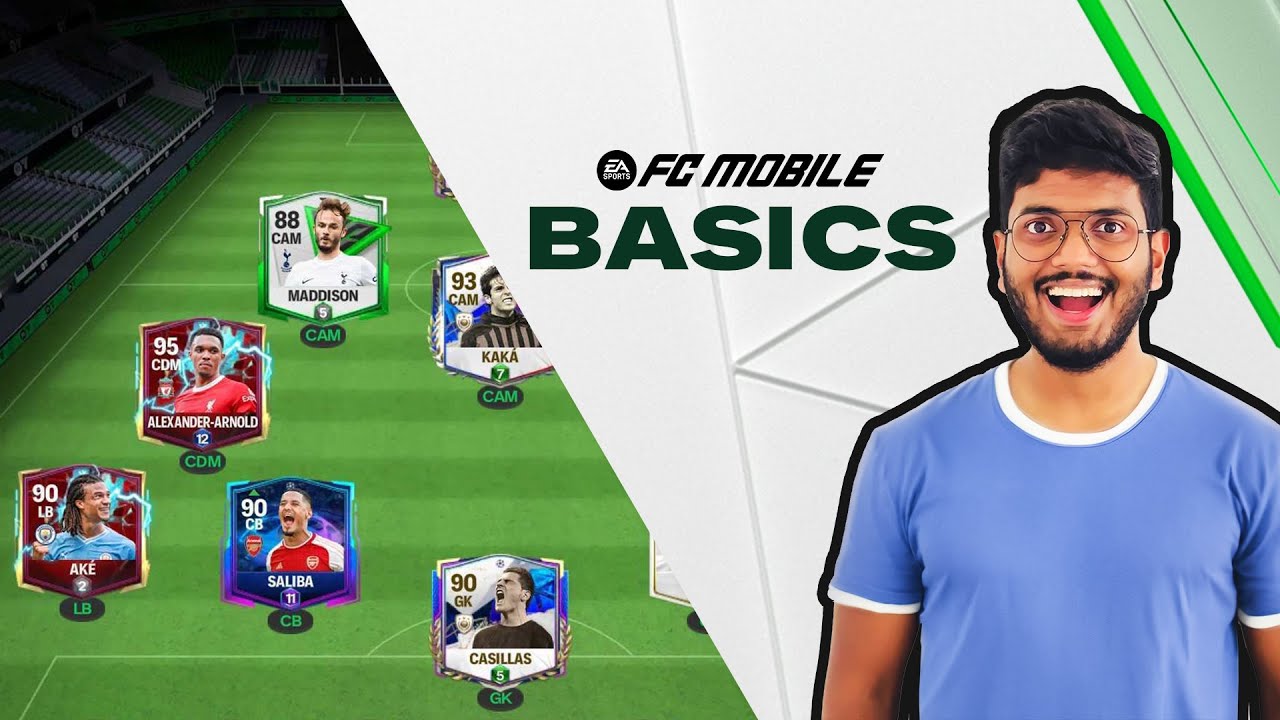
Welcome to EA SPORTS FC™ MOBILE 24 | Basics Guide